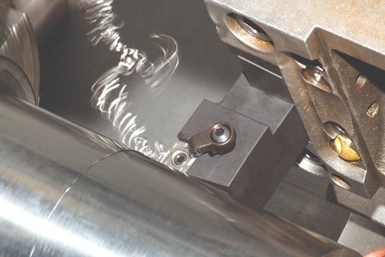
Greenleaf’s G-9610 is a PVD-coated carbide grade designed for turning titanium-based alloys. The wear-resistant, chemically stable and lubricious coating is said to protect the heat-resistant, submicron substrate and enable higher speeds and extended tool life in continuous cuts.
Photo Credits: Greenleaf Corp.Numerous difficulties inherent to turning titanium-based alloys stem from their metallurgical properties. Therefore, it is important that CNC machine shops understand such characteristics as described below before making tooling and machining choices as they endeavor to develop an effective titanium turning process. These characteristics are chemical stability, minimal heat generation/transfer, and the ability to carry varying mechanical stresses.
Titanium is an extremely potent solvent. It forms very stable bonds with oxygen, nitrogen and carbon. That means, given the right environment, titanium will rapidly degrade any areas of a cutting tool that are in contact with it that contain oxides, nitrides or carbides. All of these are elements of carbide cutting tool coatings and substrates.
Featured Content
此外,钛的热conductivity is famously low among metals. It is so low that most of the heat generated in the primary shear zone as a result of chip formation remains in the chip. The heat is then transferred to the cutting tool through the amount of contact area between the chip and the tool. Another consequence of the low thermal conductivity is that almost none of the heat that is otherwise quite useful in metal cutting is propagated ahead of the cut. In other words, none of the typical benefits (plasticization, local reduction in yield strength, lower cutting forces and reduced wear) are realized, while all of the disadvantages (softening of the cutting tool and reduced energy barrier for chemical reactions) are experienced.
Finally, titanium is very ductile and goes through a large region of elastic and plastic deformation before finally fracturing to form a chip. Once the fracture starts, elastic deformation is recovered and the deformed layer springs back, resulting in a great degree of variation in cutting forces per revolution — even in continuous cuts. Mechanically, machining titanium is likened to machining a wet sponge. Using cutting speed parameters that current tools and machining environments allow, the chip formation is cyclical and produces a segmented, serrated chip, which tends to exacerbate the mechanical abrasion of the tool.
As a result, stable and efficient turning of titanium requires selecting or designing cutting tools and machining processes to mitigate the effects of these properties. This includes careful consideration of:
Tooling selection. For rough turning, the insert substrate must have enough toughness to endure the chip formation mechanism and sufficient strength and hot hardness to maintain a sharp edge for as long as possible. Fine- or medium-grain carbide grades with low binder content are best. For finishing, the benefits of higher hot-hardness outweigh the need for elasticity and toughness meaning a submicron grade (also with low binder) would give the best results. This is exemplified inGreenleaf’s recently developed G-9610 grade, especially when paired with the TF chip form geometry, which has demonstrated high turning productivity and tool life.
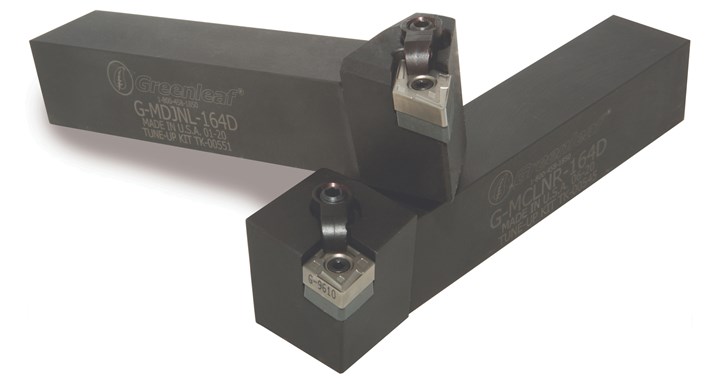
The G-9610 insert grade is said to deliver a 25% increase in material removal rate and up to 100% increase in tool life versus other grades when machining titanium. A variety of insert and chip-form geometries are available.
In addition, the insert coating needs to possess low-friction qualities to reduce heat generation and abrasive wear while preventing material deposition, known as built-up edge. It also needs to retain hardness and chemical stability at elevated temperatures, and feature an outer layer with titanium-based chemistry. Traditionally, one would not expect that the appearance of the machined material in the contact layer of the coating is a good thing, but with titanium it is a basic requirement of preventing aggressive chemical wear. TiAlN is quite popular, but PVD coatings such as those used on G-9610 are a new alternative to provide hot hardness, lubriciousness and chemical stability during titanium machining.
插入micro-geometry(磨练和土地)和宏观o-geometry (chip-forming geometry) need to perform very high shear cutting. Large negative lands or hones will generate significantly higher stresses and heat, which will drastically reduce tool life. The chip form geometry also needs to direct chip flow in such a way as to minimize the contact area between the chip and the rake face of the cutting tool and, thereby, the interface of heat transfer. The chip form geometry should be as sharp as it can be while maintaining sufficient edge strength to withstand the variation in cutting forces inherent in machining titanium at common cutting speeds (below 450 sfm). As the primary objective is reducing cutting forces and heat generation and transfer to tool, increasing flank clearance and reducing rake angles is hugely beneficial. As such, an RPGN insert will have appreciably better wear than an RCGN, despite the seemingly insignificant 4-degree difference in flank clearance.
Strain hardening (also known as work hardening) is inescapable in titanium machining. Any machining operation will leave behind a dislocation-rich surface with higher apparent hardness than the material immediately below it. Subsequent machining needs to account for this effect by making use of high-feed geometries, decreasing or increasing lead angles (depending on the convention of measurement) or, ideally, using round inserts engaged at or below a 45-degree lead to reduce the incidence of notching. Round V-bottom or RCMT-style inserts tend to be the shape of choice and are stocked with various chip forms geometries in the G-9610 grade.
Cutting conditions. The cutting speed has the greatest effect on tool life because it has the greatest effect on heat generation. In fact, increasing the feed rate is preferable to increasing the depth of cut. That’s because it produces a thicker chip, with the bulk of the heat carried within the chip shielded from transfer to the tool by the chip’s outer layers (because of titanium’s low thermal conductivity). And, while the contact area between the chip and the rake face of the cutting tool will increase as a result of increased feed rate, this relationship is not linear. Increasing the depth of cut, on the other hand, results in a proportional increase in contact area between the chip and the rake face of the cutting tool, leading to a proportional increase in heat transferred to the cutting tool. It’s best to keep the depth of cut low and instead increase the feed rate as long as the cutting forces don’t exceed the strength of the cutting tool and are well supported by the part, fixture and machine.
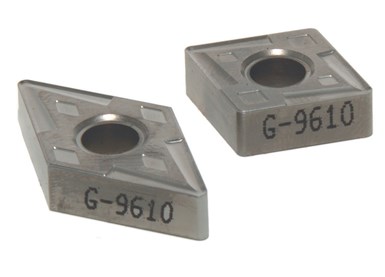
With the TF chip form geometry, it is best to run tools at higher speeds and moderate feed rates.
In addition, tool wear when machining titanium is nonlinear. As a result of wear, hones get larger, the insert coating gets thinner and the microgeometry changes shape, all of which leads to higher cutting forces and heat which accelerates wear. Wear begets more heat which begets more wear and that creates a feedback loop that, in titanium alloys, can run away quickly and lead to catastrophic failure. It is important to monitor wear and index the cutting tool before it reaches a critical point where the stresses can no longer be sustained and the tool fails.
Environmental factors. Coolant needs to be delivered in large volume and ideally at high pressure as close to the cutting edge as possible. Coolant delivered at 80 bar or higher is recommended to drive a wedge between the rake face of the cutting tool and the chip to further reduce heat transfer and abrasive wear. High-pressure or ultra-high-pressure coolant also enables increasing cutting speeds, thereby improving productivity. Inadequate coolant delivery will greatly reduce tool life.
Counter vibration by varying the cutting speed with time, within a -5% to +5% window.
The machining environment needs to be rigid and damped. If any part of the environment (part, fixture, tool or machine) responds positively to the high-frequency periodic forcing intrinsic to titanium chip formation, users will quickly run into constructive interference which, in this case, is rather destructive overall. The result can be fighting an uphill battle against vibration and all the damage that it brings, including but not limited to accelerated and irregular tool wear, poor surface quality, spindle stall, and inadequate function and accelerated wear of the guides and bearings. A general tip for countering vibration is to vary the cutting speed with time, within a -5% to +5% window. This prevents the buildup of constructive interference by continuously varying the frequency of the forcing function and should limit vibration to a minimum. A larger (±10%) window might be necessary or a smaller (±3%) window could be sufficient.
Note that while the information above is applicable to achieve clean, continuous turning of titanium-based alloys, milling or interrupted turning, or the removal of forging scale, might bring additional challenges.
RELATED CONTENT
酒吧给料机基础
Some primary factors are often overlooked when considering how to justify the implementation of a bar feeder for turning operations.
The Long And Short Of Bar Feeder Selection
For many shops, the decision comes down to a 4- or 12-foot-capacity magazine-style bar feeder. Here are some guidelines for choosing between them.
Skiving Long, Slender Parts with Tight Tolerances
Here's a look at one of the oldest and most efficient methods of screw machine production for parts that are long and slender, with close-diameter tolerances and finishes, or parts that require truly spherical radii.